Optical cross-connects - Part 1: applications and features
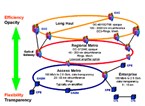
By Phillipe Perrier, Alcatel Optical Cross Connect Group
Optical networking and wavelength-based services
OXC applications
OXC features
Optical or electrical core: opaqueness or full transparency?
Cross-connects with an optical core
The dramatic growth in traffic fueled by the explosion in data communications, Internet access, mobile telephony, and the emergence of new-generation multi-media services has triggered a drastic increase in capacity requirements for metropolitan and long-haul transport networks.
The vast majority of long-haul (LH) interchange carriers have embraced wavelength division multiplexing (WDM) as the technology of choice to rapidly overcome their sudden capacity exhaust and, at the same time, to meet the network requirements of tomorrow. The reasons for selecting WDM stem from the technique's compatibility with the existing fiber infrastructure and its cost-effectiveness compared to more conventional approaches. WDM offers the potential for an enormous transmission capacity by capitalizing on the very large bandwidth of optical fibers. Products with 1.6 Tbit/s throughput on a single fiber (160 channels at 10 Gbit/s!) have recently been offered commercially by a number of equipment manufacturers (including Alcatel with its Optinex 1640), with upgrade paths to provide even more channels per fiber. To date, however, the deployment of WDM has been primarily restricted to point-to-point links, with active reconfiguration and bandwidth management performed at the synchronous optical network /synchronous digital hierarchy (SONET/SDH) layer.
Although WDM has quickly become the dominant transmission technique in long-distance communications systems, its introduction in metropolitan networks has lagged behind for a number of reasons. Unlike the homogeneous LH environment, the metro market is characterized by a complex mix of topologies, distances, and applications. Alternative solutions have allowed the local exchange carriers (LEC) to wait for maturity and cost stabilization of optical technologies. Fiber exhaust, which makes WDM an easy prove-in, is an issue in limited cases. In many instances, conduits exist to pull more fiber as a lower cost alternative to deploying WDM systems. As a consequence, WDM as simply a network infrastructure tool is therefore not as compelling for short-haul networks, and must bring more benefits to the operator to gain acceptance into the network.
Optical networking and wavelength-based services
The wide adoption of WDM in the backbone network is not only providing the required capacity expansion but is also driving the network towards the next-generation infrastructure, known as the photonic layer, a single multi-service network designed to handle growth, limit overlay networks, and improve bandwidth efficiency (see Figure 1). This layer will enable a new class of high-revenue end-to-end wavelength services for customers who want complete flexibility for their high-bandwidth data needs.
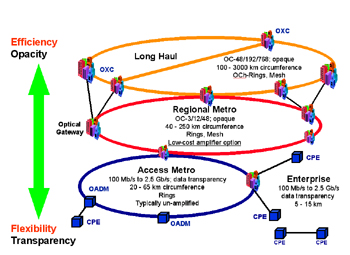
Flexibility and efficiency. With network planners now turning to optics to evolve existing point-to-point WDM links into a scalable and robust optical network, the challenge facing the industry is to offer flexibility in local networks via bit rate and protocol transparency, while maximizing bandwidth management efficiency in LH networks.
- In backbone networks, photonic technology developments will be directed at enabling more efficient wavelength grooming, routing, and network protection/restoration of high bit-rate information signals.
- The catalyst behind the use of WDM in short-haul networks may be its promise of transparency in offering new high-end wavelength-based services. Several shades of transparency have been envisioned, spanning the spectrum from full transparency (format, protocol, bit-rate) to some sub-set. Users would potentially have the flexibility to transport any kind of data without regard for the restrictions imposed by the digital hierarchy (SONET/SDH). A mixture of voice, data, and video could be carried with any protocol, and the data rate could change as needed – all under the complete control of the end-user.
Wavelength services. End-to-end wavelength services are expected to take various forms. For instance, a wavelength service could be the exchange of bandwidth between LH carriers at two major network hubs. It could also be selling an end-to-end wavelength to a Fortune 500 customer between two locations within a city, or even between cities across the world. The emergence of these services would profit both operators and customers:
- By selling wavelengths rather than dark fibers, the carrier can benefit from the large capital invested in its fiber plant without actually giving up these fiber assets to customers.
- Customer benefit by having complete control over the content and format of the information to be carried on its network without the cost of a dedicated network infrastructure.
Optical cross-connect (OXC) systems are expected to be the cornerstone of the photonic layer providing carriers more dynamic and flexible options in building network topologies with enhanced survivability. Today, such equipment exists primarily in many telecom equipment manufacturers' labs; few have been demonstrated in long-term field trials, usually as part of national programs. These optical cross-connects are poised, however, to break out of the experimental stage and find their way into all areas of the network, first in the long-haul network, at large point-of-presence (POP) sites between carriers and large hub sites within carriers, and, ultimately, in interoffice and access networks.
The main function of the OXC will be to reconfigure the network dynamically - at the wavelength level – either for restoration purposes or to accommodate changes in bandwidth demand.
To optimize service delivery in this multi-service environment (photonic layer), OXC must work in concert with a network element that will format the information in a manner that can be easily handled by OXCs. Where lower-speed networks (eg, metropolitan networks) and high-speed fixed bit-rate networks (eg, backbone) meet, optical gateways will emerge to bridge the two network types, grooming in the electrical domain lower-speed signals from metro networks into tightly packed information streams before passing it to optical cross-connects for economical transport in the long-haul network.
The optical gateway is a key network element of the optical networking vision, providing a platform with integrated colored optics, matched to the ITU-T WDM grid, while internally managing broadband services in the electrical domain. Optical gateways will displace the existing broadband digital cross-connect systems (DCS) (or the DCS will evolve to optical gateways) to manage:
- The transmission rate change between aggregation and backbone networks, in effect grooming lower-rate wavelengths from metropolitan networks to high SONET/SDH-rate ITU-compliant channels for the backbone network
- The broad range of payloads anticipated on wavelength services, simultaneously supporting cell-based routing and aggregations of ATM or IP payloads and legacy TDM services.
OXC are contemplated for:
- Connection and bandwidth management, to provision connections of clear channels (for private line wavelength services) and of optical channels (with the ability to support non-SONET/SDH payloads), as well as to provide (wavelength) add/drop functions, etc.
- Wavelength grooming, to better utilize the installed infrastructure.
- Smooth growth from 10 to 40 Gbit/s services, reducing network cost.
- Protection /restoration, at the wavelength level, for instance for unprotected router ports, to maximize the efficiency and reliability of the backbone network infrastructure at a lower network cost.
- Routing and interconnection at the wavelength level, as an automated optical distribution frame with integrated access, thus potentially displacing some fiber patch panels, as well as to manage wavelengths between interconnected rings.
- Dynamic wavelength allocation, when coupled with core routers, to achieve a cost-effective solution against fluctuating bandwidth requirements.
These applications would be offered under a unified network management system, allowing rapid end-to-end service turn-up as well as network-level service level agreement (SLA).
Number of ports. Though significant with respect to today's technological capabilities, the port count on OXCs in the backbone network is likely to be fairly small in the near term (512x512 equivalent single-wavelength ports), but growing to several thousands in the future.
Bit rates and supported protocols. As previously indicated, the backbone network will continue to support SONET/SDH frames for the next few years with signal rates restricted to 2.5 and 10 Gbit/s, with 40 Gbit/s around the corner.
Single wavelength port. In the initial deployment strategy, the OXC ports will be single wavelength. However, as the photonic layer evolves, WDM compatible ports (integrating the wavelength demultiplexing/multiplexing functions within the cross-connect) could result in significant benefits from the network management and cost point of view. This evolution would, however, require either the definition of standard multi-wavelength interfaces (not likely to happen any time soon) or a single-vendor environment.
Full connectivity, in a strictly non-blocking fashion. Any connection from an available input port to any available output port can be established without disrupting/rearranging the existing connections, and without affecting the quality of these connections in any way.
Multi-casting. Multicast connections (at least 1x2) must be supported from any input to any set of outputs, without any restriction. In particular, any number of multicast connections should be supported simultaneously. The OXC must remain strictly non-blocking under multi-casting conditions.
The combination of full connectivity and multicasting capability is of particular interest for add/drop with drop-and-continue functionality as well as for test access, enabling any subset of the OXC input/output ports to be assigned, at the user's discretion, as (single wavelength) add/drop ports or test access ports. In the latter case, the operator can then connect any port(s) to the assigned test access port(s) by establishing the appropriate connections through the switching fabric. Test access ports can then be connected directly to external equipment, allowing terminating and pass-through connections to be tested within the OXC.
Scalability. To enable growth to large sizes.
Modularity. The ability to populate the OXC as a function of required capacity would reduce the cost of installation and of future growth.
Fast switch times, for provisioning and restoration. Private lines can tolerate provisioning times in the order of a second. Regarding restoration, the SONET/SDH target is 50 ms target, while current meshed networks can require as much as several minutes. It is expected that most applications would be satisfied with sub-second restoration.
Survivability, through full (1 1) redundancy of the switching fabric and of the control platform. The dual copy architecture will also warrant efficient and low-risk in-service upgrade without affecting traffic.
Operation, administration, maintenance, and provisioning. The OXC must provide connection verification and monitoring capabilities.
Arguably, the main barrier to the introduction of optical cross-connects lies in the area of network management. Legacy operations systems (OS) did not contemplate this wavelength management functionality. New capabilities must be driven into the network elements and element managers to maintain control and interconnect with existing networks. Few operators have the expertise to operate WDM systems, let alone OXCs, and few standards exist on how to best to manage a wavelength circuit through a network.
In the near term, vendors will supply proprietary management systems to solve these problems. However, agreements within the industry must be reached regarding the type of information to be monitored, the technique used to create the optical service channels, and the way in which these channels are monitored and controlled with large operator management systems. Newer players in the telecommunication industry may be able to implement these new services sooner as they are not encumbered by large legacy management systems.
Optical or electrical core: opaqueness or full transparency?
Optical or electrical core? So far, building a scalable optical switching fabric has proved difficult because of a lack of large optical space switch building blocks. This limitation has had a direct impact on possible OXC architectures, ultimate size, and cost, and has led some equipment manufacturers to propose an optical layer cross-connect based on an electrical matrix. As a result, most optical cross-connects advertised today in the industry have electronics - not optics - at their core and process signals for which the maximum bit rate is 2.5 Gbit/s (Figure 2a).
The reasoning is that since the optical signals entering and leaving the OXC are going through optical-to-electrical (O/E) interfaces anyway (see next section), why not also perform the switching function electronically? The argument is valid, especially from a cost perspective. However, it starts to fail as bit rates increase in LH networks.
- As of now, 10 and 40 Gbit/s electrical cross-points are not commercially available. New materials, such as silicon germanium (SiGe), which would allow 10 Gbit/s operation, are under investigation. Initial results, however, indicate that switching devices based on these technologies will be restricted to fairly small sizes, maybe as small as 32x32, before impairments such as crosstalk and power consumption become too complex to resolve. A multi-stage architecture would thus be required to implement very high-port count cross-connects.
If current ultra-band cross-connects (2.5 Gbit/s electrical switch fabric) were to be used in the backbone network, where the preferred transmission rate is rapidly evolving from 2.5 Gbit/s to 10 Gbit/s, four 2.5 Gbit/s ports would have to be used to handle each 10 Gbit/s signal, in effect reducing the cross-connect size by a factor of 4. The same scheme would have to be repeated to handle higher rate signals (such as 40 Gbit/s), further reducing the size of the cross-connects.
- Electrical interconnection of electronic switch building blocks in a multi-stage architecture will become increasingly complex (to bridge the interconnection distances, to overcome electro-magnetic interference, etc), cumbersome (heavy, relatively large diameter cables), and expensive, the higher the bit rate and the larger the cross-connect size. Although electrical switching blocks at 10 Gbit/s are expected to be cheaper than their optical counterparts, economic models tell us that an overall system based on an electrical core with electrical interconnections would be more expensive.
Optical interconnection is then an attractive (if not the only) alternative to overcome these limitations. However, if the interconnection between the switch ICs is optical, wouldn't it make sense to also realize the switching function optically, thus saving on the number of O/E/O interfaces internal to the fabric?
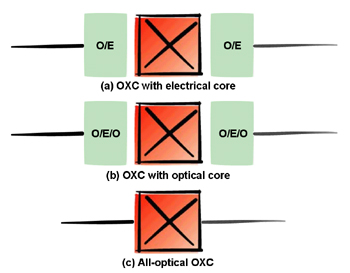
As a consequence, cross-connects with an optical, instead of an electrical, core (see figures 2b and 2c) have been proposed. Although an all-optical cross-connect would be an attractive solution (see Figure 2c) from a cost (no input/output O/E/O conversion) and transparency point of view, we expect that an opaque solution, based on an optical fabric surrounded by 3R function (see Figure 2b) will first be deployed, for the following reasons:
Opaqueness or full-transparency. In our optical network model where the backbone is optimized for bulk transport efficiency, LH networks will continue to support SONET/SDH payloads for a few more years. Transparency to the protocol is therefore not an immediate issue in this part of the network. Payload rates will be limited to OC-48/STM-16, OC-192/STM-64 (with OC-768/STM-256 around the corner). Indeed, it is unlikely that a long-distance carrier, wishing to operate its wavelengths to the fullest with the highest bit-rate possible, will be willing to consume a wavelength for a service terminating through a local network using only a few Mbit/s.
Bit-rate transparency over the supported set of rates would greatly enhance network flexibility. The drawback of this approach is that the span must be engineered for the worst case (ie, highest bit-rate), potentially driving up the cost of the network. Thus, 3R regeneration, whether single- or multi-rate, remains a key function of the backbone network and its key cost component. This domination will grow as the channel count and the per-channel rate increase. Although promising results have been obtained on optical regeneration, optoelectronic (O/E/O) regeneration remains the most efficient and cost effective implementation today.
Full (or analog) transparency at this level of the network would "future proof" the infrastructure against bit-rate increase and new traffic types, and would minimize the equipment in the signal path, resulting in significant cost advantage. However, this same transparency does limit the scalability (in terms of the number of channels, bit-rate, etc) or the geographical extent of the network. These limitations arise from:
- The accumulation of transmission impairments, resulting from chromatic dispersion (CD; see Understanding and measuring chromatic dispersion polarization mode dispersion (PMD), non-linear effects (NLE), component crosstalk, amplifier noise, gain curvature, polarization dependent loss (PDL), wavelength misalignment, filter narrowing and mis-alignment.
- The difficulty in engineering the network, which would have to be engineered for the "worst case". The worst path (for example, the longer restoration path) would thus have to be known from the outset; all components would need to be specified for this worst path, and so on. Furthermore, transparency requires that the whole network be engineered at the same time. Once engineered, the network can not be extended beyond its intended design limits.
To overcome these limitations, the deployment of cross-connects with an optical core is expected to begin with standard, open, O/E/O interfaces. These offer a number of advantages:
- Facilitate the growth of the photonic layer by preventing impairments from accumulating and by providing wavelength translation for free
- Boost the introduction of optical routing/switching in the photonic layer with current WDM systems
- Facilitate fault location and performance monitoring (such as bit error rate monitoring; analog measurements, like optical signal-to-noise ratio, are insufficient), in effect simplifying network management
- Allow multi-vendor interoperability, alleviating the lack of standard transparent interfaces and ensuring signal quality assurance hand-shaking between vendors
- Facilitate the adoption of new technologies as they become available.
As a result, a central office (CO) of the photonic layer will look, for the near term, as illustrated in Figure 3.
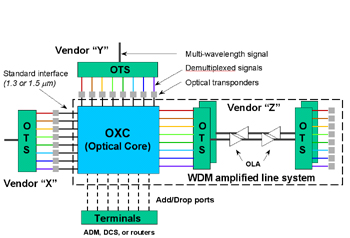
With maturing technologies, the photonic layer is then expected to evolve to include "islands" of transparency, where transparent subnetworks of limited extent would be interconnected via opaque interfaces. The concept is relatively simple and attractive. Given that the network engineering grows in complexity as the network gets larger, restricting the scale of the network (in terms of distance, number of node, etc) will make the problem easier to solve.
This concept could potentially result in significant cost savings, in particular for systems with large wavelength counts and high through/drop ratios at nodes internal to the sub-network. However, and as often is the case, the optimal extent of transparency will ultimately be determined by cost / performance tradeoffs.
Cross-connects with an optical core
Three broad classes of optical cross-connects (see Figure 4) have been defined today (Telcordia's Optical Cross-Connect Generic Requirements, GR-3009-CORE):
- Fiber switch cross-connect (FXC)
- Wavelength selective cross-connect (WSXC)
- Wavelength interchanging cross-connect (WIXC).
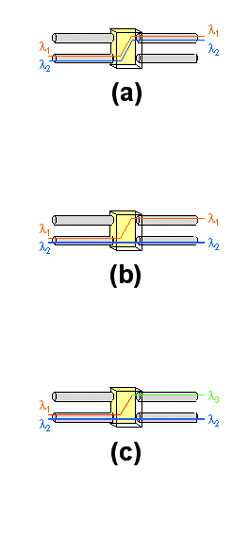
A fiber switch cross-connect (see Figure 4a) switches all of the wavelength channels from one input fiber to an output fiber, in effect acting as an automated fiber patch panel. FXC are less complex (and thus expected to be less expensive) than a wavelength selective or wavelength interchanging cross-connect. In parts of the network where protection against fiber cuts is the main concern, FXCs could be a viable solution. They may also make the best use of current proven optical technologies. While FXCs can provide simple provisioning and restoration capabilities, they may not offer the flexibility required to promote new end-to-end wavelength generating services.
A wavelength selective (see Figure 4b) cross-connect can switch a subset of the wavelength channels from an input fiber to an output fiber. Functionally, they therefore require demultiplexing (in the frequency domain) of an incoming wavelength multiplex into its individual constituent wavelengths. This type of cross-connect offers much more flexibility than an FXC, allowing the provisioning of wavelength services, which in turn can support video distribution, distance learning, or a host of other services. A WSXC also offers better flexibility for service restoration; wavelength channels can be protected individually using a mesh, ring or hybrid protection scheme.
A wavelength interchanging cross-connect (see Figure 4c) is a WSXC with the added capability to translate or change the frequency (or wavelength) of the channel from one frequency to another. This feature reduces the probability of not being able to route a wavelength from an input fiber to an output fiber because of wavelength contention. WIXC offer the greatest flexibility for the restoration and provisioning of services. When shopping for a WIXC, however, read the fine print; not all WIXC are equal. Based on the technology used to implement the wavelength conversion function, it is sometimes difficult for a cross-connect to convert a channel at a given "color" to that same "color". Remember that a WISX is also expected to handle channels for which wavelength is to be left unchanged, and thus should be able to "translate" to the same wavelength.
Optical Cross-Connects – Part 2: enabling technologies discusses the different optical switching technologies and evaluates their strengths and weaknesses.
About the author…
Phillipe Perrier is product manager for the Alcatel Optical Cross Connect Group, Paris, France.